What are the best ways to decarbonize aviation?
What are the best ways to decarbonize aviation?
Across the globe, governments, companies and industries are mobilizing to accelerate the net-zero transition. In all sectors of the economy, from steel manufacturing to banking and aviation, innovative technologies are being deployed to decarbonize the global economy to meet ambitious climate targets such as those included in the Paris Agreement. This is no small task, especially when it comes to aviation, which currently contributes approximately 2% of global carbon emissions, equivalent to 800 million tons of carbon dioxide annually (2022).
The vast majority of these emissions come from burning conventional jet fuel in jet engines. While 2% may not seem like a high number, those emissions are very challenging to reduce given the limited low-carbon options currently available to the industry and the growing global demand for air travel. In addition, while other sectors of the economy continue to decarbonize, aviation emissions are projected to increase as air travel continues to grow. Flying has become essential for personal lives, work, and the global economy. The reality is we are not going to stop flying and for most people, alternatives to air travel are hard to come by—but do greener alternatives exist?
Expert consultants, researchers and organizations such as the International Civil Aviation Organization (ICAO) typically discuss three alternatives to traditional jet fuel to reduce aviation’s carbon emissions: sustainable aviation fuel (SAF), hydrogen, and electrification (batteries). While all of these options are theoretically feasible, some are better than others, particularly in the near term. This topic can be pretty tricky to wrap your head around, so we pulled a list of pros and cons for each alternative and suggested five pillars for analyzing alternatives to traditional jet fuel, including:
01
Greenhouse gas emissions reductions
02
Density and weight
03
Supply chain implications
04
Price parity with incumbent fuels
05
Long-haul flight capabilities (As shown in Table 1 below)
A few things to note: green hydrogen can be leveraged as both a direct fuel for hydrogen aircraft and as a feedstock for a liquid fuel (eSAF); meanwhile electric planes would require a unique battery with high energy density while being light and compact enough to fly passenger planes.
Our Pillars
P1
The first pillar underscores the goal of this undertaking—to significantly reduce carbon emissions to mitigate climate change. For example, on the SAF side, the most common SAF in use today which is known as “HEFA” SAF, can achieve ~70-100% GHG reductions when using waste feedstocks (30-60% when using first generation feedstocks), while synthetic SAFs made from biogenic CO2 (such as ours), can be carbon neutral, reducing a larger amount of greenhouse gas emissions. Similarly, hydrogen and electric aircraft both demonstrate deep emissions reductions potential.
P2
The second pillar—matching fuel density and weight—is rooted in physics. In simple terms, fuels and batteries have different densities that make them better or more efficient for air travel. For example, traditional jet fuel has an energy density equal to 43 MJ/kg, while lithium-ion batteries in today’s electric vehicles have an energy density equal to 0.72 MJ/kg. Batteries’ low energy density severely limits opportunities for electrification at this time. While batteries continue to evolve, they still need significant improvements to pack enough energy in a small space and be light enough for lift-off—about 35 tons of batteries would be needed to power an aircraft the size of a Boeing 737. The reality is that current battery limitations lead to a lack of range and weight challenges, making them impractical for large passenger aircraft travel.
P3
Pillar three provides more context on the logistics of these alternatives. It explores aviation's current supply chain and how it would be altered with greener alternatives. For example, when it comes to hydrogen as fuel for hydrogen planes, an entirely new supply chain would be needed, with infrastructure surrounding hydrogen refueling stations. The same would apply to the electrification route, with improvements needed in battery efficiencies, as well as for aircraft design and airport infrastructure. On the other hand, SAF is the short, medium and potentially even long-term green alternative that is much more compatible with aviation’s current infrastructure. For example, AIRMADE® SAF is being designed to be a “drop-in” fuel that can be used in existing planes and refueling infrastructure without modifications and can directly displace conventional and carbon-intensive jet fuel.
P4
Pillar four looks at the economics of each alternative. Airlines already have some of the smallest profit margins at around 3% and according to the International Air Transport Association (IATA), the average profitability per ticket falls around ~$2.60. With such slim margins, a green premium associated with SAFs makes it challenging for airlines to pay for cleaner alternatives. Without government support or innovative private sector mechanisms (e.g., Book and Claim), which are discussed more thoroughly below, these costs may fall on the consumer.
P5
Finally, the fifth pillar touches on flight duration or the requirement for alternatives to be compatible with long (8-16 hours) and ultra-long flights (16+ hours). In other words, airlines need to ensure that the new technology is compatible with long flight times, without sacrificing cost, time, or weight. Generally, aircraft are filled up with enough fuel for one leg of their flight (plus emergency fuel), to keep them as light as possible. In the future, greener alternatives will need to do the same.
Taking a holistic approach, we put together this matrix to summarize the pros and cons of each pathway, leveraging industry knowledge, research, and in-house expertise to rate them as low, medium or high feasibility of adoption compared to conventional jet fuel.
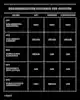
Sustainable Aviation Fuel (SAF)
P1
GHG Reductions: High
SAFs can be produced from a number of sources (feedstocks) including waste plant oil and fats, green and municipal waste, non-food crops, as well as hydrogen and CO2. In order to be an eligible SAF under ICAO CORSIA, it must have GHG emissions that are at least 10% below traditional jet fuel (89 gCO2e/MJ). In some cases, eSAF that leverages biogenic CO2 (like AIRMADE® SAF) can be considered carbon neutral.
P2
Density & Weight: Medium
SAFs typically meet or exceed the necessary fuel density for flights of 43 MJ/kg, but are less efficient than batteries.
P3
Supply Chain Logistics: Medium
SAF can be a drop-in fuel option, but most SAFs still require blending with conventional jet fuel to work in existing aircraft. Furthermore, regulatory requirements mandate a 10-50% blending threshold. Blending ensures that there is enough “lubricant” or aromatics in the fuel to ensure safe aircraft operations.
P4
Parity with Jet Fuel: Low
Price parity to incumbent fuels is a challenge but jurisdictions are adopting policies to help reduce the green premium. U.S. jet fuel currently retails at around $2.85 per gallon while SAF prices are at $6.69 per gallon. Thus, innovative financial mechanisms are needed to accelerate SAF uptake.
P5
Flight Duration Compatibility: High
SAF is suitable for long-haul flights.
Hydrogen
P1
GHG Reductions: High
H2 has zero direct carbon emissions and includes some co-benefits. Additional non-CO2 benefits and disadvantages are still being researched, but preliminary findings indicate that there are moderate benefits for NOx and contrails as by-products.
P2
Density & Weight: Medium
Hydrogen is the lightest element, but it has a much lower energy density than kerosene, meaning aircraft powered by it instead of fossil fuels would actually weigh more. To combat volumetric challenges, H2 has to be stored in liquid form, which means installing high pressure tanks on planes that keep the highly flammable substance at temperatures below -253 Celsius. All that adds weight, cutting into range, cargo, and passenger capacity.
P3
Supply Chain Logistics: Low
Unlike kerosene fuel, hydrogen does not currently have an established supply chain in aviation. Additional concerns such as safety (in production, handling, and use), and infrastructure to implement and store liquid hydrogen pose challenges.
P4
Price Parity with Jet Fuel: Low
Price parity to incumbent fuels is a challenge, especially because the cost of hydrogen liquefaction and storage is very high (can add up to $2.75/kg to the total cost). Moreover, it would require a new fleet of airplanes that are compatible with liquid H2.
P5
Flight Duration Compatibility: Medium
Long-haul flights may be challenging in the foreseeable future, but medium and short ones are feasible. Considering current aircraft designs, hydrogen aircraft could be range limited to up to 2,500 kilometers. Hydrogen aircraft designs currently exist (i.e. ZeroAvia).
Electrification
P1
GHG Reductions: High
Planes powered by batteries have zero direct carbon emissions, reduce noise, and cause no contrails.
P2
Density & Weight: Medium
Batteries are efficient in how they use electricity. In an electric plane, about 70% of the energy used to charge up a battery would actually power the plane. There are some losses in the battery and in the motor, but the efficiency is high compared to hydrogen and SAF. However, the added weight and large size of the batteries make them a difficult switch.
P3
Supply Chain Logistics: Low
Electrifying planes would involve changing every aspect of the supply chain, from the planes themselves to the airport infrastructure.
P4
Price Parity with Jet Fuel: Low
Electric vertical-takeoff-and-landing vehicles (eVTOLs) would require extremely fast high-power charging or rapid battery swapping, which can be quite cost intensive.
P5
Flight Duration Compatibility: Low
Battery technology is not advanced enough to support medium or long-haul flights.
Looking at the bigger picture, SAF is the most technically feasible solution in the short, medium and potentially long term. While it currently is not at price parity with conventional jet fuel, it can offer significant greenhouse gas emissions reductions, fuel density improvements, drop-in capabilities and suitability for long-haul flights. SAF has also become of interest to corporations looking to reduce their Scope 3 emissions, which tend to come from business travel and other downstream activities. For example, in the past several years, large companies like Boston Consulting Group (BCG) and Microsoft have gone public with commitments to purchase SAF. While these commitments will be realized within the next few years, they show growing support for a pathway to large-scale aviation emissions abatement.
We also see this growing momentum for aviation decarbonization in the global policy landscape. The UK SAF Mandate, which takes effect in 2025, requires that 10% of all jet fuel in flights taking off from the UK come from sustainable sources by 2030 and 22% by 2040. Similarly, in the European Union, ReFuelEU Aviation aims to increase both demand for and supply of SAF to put air transport on the trajectory of the EU’s climate targets for 2030 and 2050.
Finally, the U.S. SAF Grand Challenge creates a goal of supplying sufficient SAF to meet 100% of aviation fuel demand by 2050. Within these policy mechanisms, there is also a greater emphasis on “e-fuels,” like AIRMADE® SAF, because of their deep GHG reduction potential (e.g., the UK SAF Mandate has a PtL obligation, starting at 0.5% in 2030, increasing to 1.5% in 2035, and reaching 3.5% by 2040). While mandates and targets are designed to drive SAF uptake, government incentives (e.g., Inflation Reduction Act SAF tax credit) are also being introduced to support supply and reduce production costs.
Therefore, while the full commercialization of SAF still has some hurdles to overcome, it’s promising that in Bloomberg’s most recent New Energy Outlook, they forecasted SAF consumption levels to hit 88 billion gallons per year by mid-century. In addition to SAF deployment, jet-fueled aircraft could still gain 15-20% in terms of efficiency compared to the best technology available today, which will also support larger decarbonization goals. Ultimately, our mission at AIR COMPANY is to support climate mitigation on a large scale, with smart, effective and cutting-edge technologies at the forefront of this transformation. We’re confident that SAF will play an integral role in this transition today and into the future.